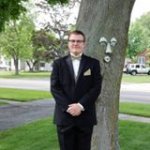
The reason why something like inflation was needed in cosmology was highlighted by discussions of two key problems in the 1970s. The first of these is the horizon problem -- the puzzle that the Universe looks the same on opposite sides of the sky (opposite horizons) even though there has not been time since the Big Bang for light (or anything else) to travel across the Universe and back. So how do the opposite horizons "know" how to keep in step with each other? The second puzzle is called the flatness problem This is the puzzle that the spacetime of the Universe is very nearly flat, which means that the Universe sits just on the dividing line between eternal expansion and eventual recollapse.
Ever since 1905, when Albert Einstein revealed his special theory of relativity to the world, the speed of light has had a special status in the minds of physicists. In a vacuum, light travels at 299 792 458 meters per second, regardless of the speed of its source. There is no faster way of transmitting information. It is the cosmic speed limit. Our trust in its constancy is reflected by the pivotal role it plays in our standards of measurement. We can measure the speed of light with such accuracy that the standard unit of length is no longer a sacred meter bar kept in Paris but the distance traveled by light in a vacuum during one 299 792 458th of a second. So I ask?
Why do opposite sides of the universe look the same?
It's a puzzle, you see, because the extremes of today's visible universe should never have been in touch. Even back in the early moments of the big bang, when these areas were much closer together, there wasn't enough time for light - or anything else - to travel from one to another. There was no time for temperature and density to get evened out; and yet they are even. One solution: light used to move much faster. But to make that work could mean a radical overhaul of Einstein's theory of relativity.
During inflation the Universe expanded a factor of 1054, so that our horizon now only sees a small piece of what was the total Universe from the Big Bang.
The cause of the inflation era was the symmetry breaking at the GUT unification point. At this moment, spacetime and matter separated and a tremendous amount of energy was released. This energy produced an overpressure that was applied not to the particles of matter, but to spacetime itself. Basically, the particles stood still as the space between them expanded at an exponential rate.
Note that this inflation was effectively at more than the speed of light, but since the expansion was on the geometry of the Universe itself, and not the matter, then there is no violation of special relativity. Our visible Universe, the part of the Big Bang within our horizon, is effectively a `bubble' on the larger Universe. However, those other bubbles are not physically real since they are outside our horizon. We can only relate to them in an imaginary, theoretical sense. They are outside our horizon and we will never be able to communicate with those other bubble universes.
Inflation solves the flatness problem because of the exponential growth. Imagine a highly crumbled piece of paper. This paper represents the Big Bang universe before inflation. Inflation is like zooming in of some very, very small section of the paper. If we zoom in to a small enough scale the paper will appear flat. Our Universe must be exactly flat for the same reason, it is a very small piece of the larger Big Bang universe.
The horizon problem is also solved in that our present Universe was simply a small piece of a larger Big Bang universe that was in causal connection before the inflation era. Other bubble universes might have very different constants and evolutionary paths, but our Universe is composed of a small, isotropic slice of the bigger Big Bang universe.
- Read more...
- 0 comments
- 2,496 views