Regents Physics - Electrostatics
Coulomb's Law

We know that like charges repel, and opposite charges attract. In order for charges to repel or attract, they apply a force upon each either. Similar to the manner in which the force of attraction between two masses is determined by the amount of mass and the distance between the masses, as described by Newton's Law of Universal Gravitation, the force of attraction or repulsion is determined by the amount of charge and the distance between the charges. The magnitude of the electrostatic force is described by Coulomb's Law.
Coulomb's Law states that the magnitude of the electrostatic force (Fe) between two objects is equal to a constant, k, multiplied by each of the two charges, q1 and q2, and divided by the square of the distance between the charges (r2). The constant k is known as the electrostatic constant, and is given on the reference table as: .

Notice how similar this formula is to the formula for the gravitational force! Both Newton's Law of Universal Gravitation and Coulomb's Law follow the inverse-square relationship, a pattern that repeats many times over in physics. The further you get from the charges, the weaker the electrostatic force. If you were to double the distance from a charge, you would quarter the electrostatic force on a charge.
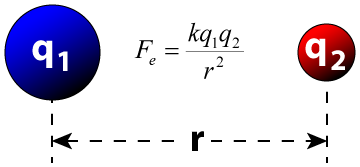
Formally, a positive value for the electrostatic force indicates that the force is a repelling force, while a negative value for the electrostatic force indicates that the force is an attractive force. Because force is a vector, you must assign a direction to it. To determine the direction of the force vector, once you have calculated its magnitude, use common sense to tell you the direction on each charged object. If the objects have opposite charges, they are being attracted, and if they have like charges, they must be repelling each other.
Question: Three protons are separated from a single electron by a distance of 1*10-6 m. Find the electrostatic force between them. Is this force attractive or repulsive?
Answer:
Electric Fields
Also similar to gravity, the electrostatic force is a non-contact force. Charged objects do not have to be in contact with each other to exert a force on each other. Somehow, a charged object feels the effect of another charged object through space. The property of space that allows a charged object to feel a force is a concept called electric field. Although we cannot see an electric field, we can detect its presence by placing a positive test charge at various points in space and measuring the force the test charge feels.
While looking at gravity, the gravitational field strength was the amount of force obsered by a mass per unit mass. The electric field strength is the amount of electrostatic force observed by a charge per unit charge. Therefore, the electric field strength, E, is the electrostatic force observed at a given point in space divided by the test charge itself. Electric field strength is measured in Newtons per Coulomb (N/C).

Electric Field Lines
Since we can't actually see the electric field, we can draw electric field lines to help us visualize the force a charge would feel if placed at a specific position in space. To help us visualize the electric field, we can draw electric field lines in space. These lines show the direction a positive charged particle would feel a force if it were placed at that point in space. The more dense the lines are, the stronger the force a charged particle would feel, therefore the stronger the electric field. As the lines get further apart, the strength of the electric force a charged particle would feel is smaller, therefore the electric field is smaller.
By convention, we draw electric field lines showing the direction of force on a positive charge. Therefore, to draw electric field lines for a system of charges, follow these basic rules:
- Electric field lines point away from positive charges, and toward negative charges.
- Electric field lines never cross.
- Electric field lines always intersect conductors at right angles to the surface.
- Stronger fields have closer lines.
- Field strength and line density decreases as you move away from the charges.
Let's take a look at a few examples of electric field lines, starting with isolated positive (left) and negative (right) charges. Notice that for each charge, the lines radiate outward or inward spherically. The lines point away from the positive charge, since a positive test charge placed in the field (near the fixed charge) would feel a repelling force. The lines point in toward the negative fixed charge, since a positive test charge would feel an attractive force.
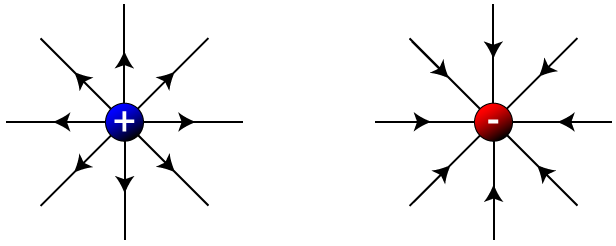
If you have both positive and negative charges in close proximity, you follow the same basic procedure:
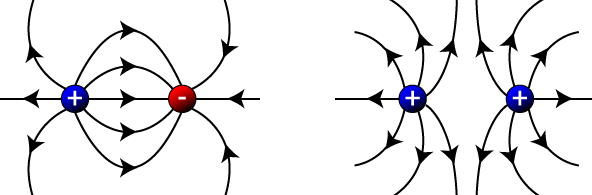
Of course, electric field lines actually lie in three dimensions, as demonstrated in this video animation.
Comparing Electrostatics & Gravity
Because gravity and electrostatics have so many similarities, let's take a minute to do a quick comparison of electrostatics and gravity.
Electrostatics
Force:
Field Strength:
Field Strength:
Electrostatic Constant:
Charge Units: Coulombs
Gravity
Force:
Field Strength:
Field Strength:
Gravitational Constant:
Mass Units: kilograms
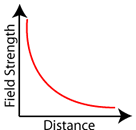
The big difference between electrostatics and gravity? The gravitational force can only attract, while the electrostatic force can both attract and repel. Notice again that both the electric field strength and the gravitational field strength follow the inverse-square law relationship. Field strength is inversely related to the square of the distance.
Electric Potential Difference
When we lifted an object against the force of gravity by applying a force over a distance, we did work to give that object gravitational potential energy. The same concept applies to electric fields as well. If you move a charge against an electric field, you must apply a force for some distance, therefore you do work and give it electrical potential energy. The work done per unit charge in moving a charge between two points in an electric field is known as the electric potential difference, (V). The units of electric potential are volts, where a volt is equal to 1 Joule per Coulomb. Therefore, if you did 1 Joule of work in moving a charge of 1 Coulomb in an electric field, the electric potential difference between those points would be 1 volt. This is given to you in the reference table as:

V in this formula is potential difference (in volts), W is work or electrical energy (in Joules), and q is your charge (in Coulombs). Let's take a look at a sample problem.
Question: A potential difference of 10.0 volts exists between two points, A and B, within an electric field. What is the magnitude of charge that requires 2.0 × 10–2 joule of work to move it from A to B?
Answer:
When dealing with electrostatics, often times the amount of electric energy or work done on a charge is a very small portion of a Joule. Dealing with such small numbers is cumbersome, so physicists devised an alternate unit for electrical energy and work that can be more convenient than the Joule. This unit, known as the electron-volt (eV), is the amount of work done in moving an elementary charge through a potential difference of 1V. One electron-volt, therefore, is equivalent to one volt multiplied by one elementary charge (in Coulombs): 1 eV = 1.6*10-19 Joules.
Question: A proton is moved through a potential difference of 10 volts in an electric field. How much work, in electron-volts, was required to move this proton?
Answer:
Parallel Plates
If you know the potential difference between two parallel plates, you can easily calculate the electric field strength between the plates. As long as you're not near the edge of the plates, the electric field is constant between the plates, and its strength is given by:

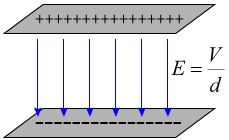
You'll note that with the potential difference V in volts, and the distance between the plates in meters, units for the electric field strength are volts per meter [V/m]. Previously, we stated that the units for electric field strength were newtons per Coulomb [N/C]. It is easy to show that these units are equivalent:
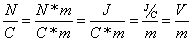
Question: Which electrical unit is equivalent to one joule?
- volt / meter
- ampere * volt
- volt / Coulomb
- Coulomb*volt
Answer: (4) Coulomb*volt
Let's try another sample problem:
Question: The diagram represents two electrons, e1 and e2, located between two oppositely charged parallel plates. Compare the magnitude of the force exerted by the electric field on e1 to the magnitude of the force exerted by the electric field on e2.
Answer: The force is the same because the electric field is the same for both charges, as the electric field is constant between two parallel plates.
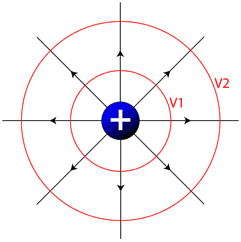
Equipotential Lines
Much like looking at a topographic map which shows you lines of equal altitude, or equal gravitational potential energy, we can make a map of the electric field and connect points of equal electrical potential. These lines, known as equipotential lines, always cross electrical field lines at right angles, and show positions in space with constant electrical potential. If you move a charged particle in space, and it always stays on an equipotential line, no work will be done.